Biochemistry is closely related to molecular biology, the study of the molecular mechanisms by which genetic information encoded in DNA is able to result in the processes of life.[ Depending on the exact definition of the terms used, molecular biology can be thought of as a branch of biochemistry, or biochemistry as a tool with which to investigate and study molecular biology.
Much of biochemistry deals with the structures, functions and interactions of biological
macromolecules, such as
proteins,
nucleic acids,
carbohydrates and
lipids, which provide the structure of cells and perform many of the functions associated with life.
[6] The chemistry of the cell also depends on the reactions of smaller
molecules and
ions. These can be
inorganic, for example
water and
metal ions, or
organic, for example the
amino acids, which are used to
synthesize proteins.
[7] The mechanisms by which cells harness energy from their environment via chemical reactions are known as
metabolism. The findings of biochemistry are applied primarily in
medicine,
nutrition, and
agriculture. In medicine, biochemists investigate the causes and
cures of
diseases.
[8] In nutrition, they study how to maintain health wellness and study the effects of
nutritional deficiencies.
[9] In agriculture, biochemists investigate
soil and
fertilizers, and try to discover ways to improve crop cultivation, crop storage and
pest control.
At its broadest definition, biochemistry can be seen as a study of the components and composition of living things and how they come together to become life, in this sense the history of biochemistry may therefore go back as far as the ancient Greeks.[10] However, biochemistry as a specific scientific discipline has its beginning sometime in the 19th century, or a little earlier, depending on which aspect of biochemistry is being focused on. Some argued that the beginning of biochemistry may have been the discovery of the first enzyme, diastase (today called amylase), in 1833 by Anselme Payen,[11] while others considered Eduard Buchner's first demonstration of a complex biochemical process alcoholic fermentation in cell-free extracts in 1897 to be the birth of biochemistry.[12][13] Some might also point as its beginning to the influential 1842 work by Justus von Liebig, Animal chemistry, or, Organic chemistry in its applications to physiology and pathology, which presented a chemical theory of metabolism,[10] or even earlier to the 18th century studies on fermentation and respiration by Antoine Lavoisier.[14][15] Many other pioneers in the field who helped to uncover the layers of complexity of biochemistry have been proclaimed founders of modern biochemistry, for example Emil Fischer for his work on the chemistry of proteins,[16] and F. Gowland Hopkins on enzymes and the dynamic nature of biochemistry.[17]
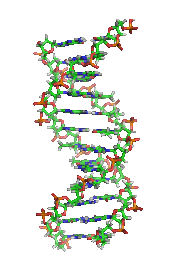
It was once generally believed that life and its materials had some essential property or substance (often referred to as the "vital principle") distinct from any found in non-living matter, and it was thought that only living beings could produce the molecules of life.[25] Then, in 1828, Friedrich Wöhlerpublished a paper on the synthesis of urea, proving that organic compounds can be created artificially.[26] Since then, biochemistry has advanced, especially since the mid-20th century, with the development of new techniques such as chromatography, X-ray diffraction, dual polarisation interferometry, NMR spectroscopy, radioisotopic labeling, electron microscopy, and molecular dynamics simulations. These techniques allowed for the discovery and detailed analysis of many molecules and metabolic pathways of the cell, such as glycolysis and the Krebs cycle (citric acid cycle), and led to an understanding of biochemistry on a molecular level.
Biomolecules[edit]
Carbohydrates[edit]
The function of carbohydrates includes energy storage and providing structure.
Sugars are carbohydrates, but not all carbohydrates are sugars. There are more carbohydrates on Earth than any other known type of biomolecule; they are used to store energy and
genetic information, as well as play important roles in cell to
cell interactions and
communications.

In these cyclic forms, the ring usually has
5 or
6 atoms. These forms are called
furanoses and
pyranoses, respectively — by analogy with
furan and
pyran, the simplest compounds with the same carbon-oxygen ring (although they lack the
double bonds of these two molecules). For example, the aldohexose
glucose may form a hemiacetal linkage between the hydroxyl on carbon 1 and the oxygen on carbon 4, yielding a molecule with a 5-membered ring, called
glucofuranose. The same reaction can take place between carbons 1 and 5 to form a molecule with a 6-membered ring, called
glucopyranose. Cyclic forms with a 7-atom ring called
heptoses are rare.
When a few (around three to six) monosaccharides are joined, it is called an
oligosaccharide (
oligo- meaning "few"). These molecules tend to be used as markers and
signals, as well as having some other uses.
[36] Many monosaccharides joined together make a
polysaccharide. They can be joined together in one long linear chain, or they may be
branched. Two of the most common polysaccharides are
cellulose and
glycogen, both consisting of repeating glucose
monomers. Examples are
cellulose which is an important structural component of plant's
cell walls, and
glycogen, used as a form of energy storage in animals.
Sugar can be characterized by having
reducing or non-reducing ends. A
reducing end of a carbohydrate is a carbon atom that can be in equilibrium with the open-chain
aldehyde(
aldose) or keto form (
ketose). If the joining of monomers takes place at such a carbon atom, the free hydroxy group of the
pyranose or
furanose form is exchanged with an OH-side-chain of another sugar, yielding a full
acetal. This prevents opening of the chain to the aldehyde or keto form and renders the modified residue non-reducing. Lactose contains a reducing end at its glucose moiety, whereas the galactose moiety forms a full acetal with the C4-OH group of glucose.
Saccharose does not have a reducing end because of full acetal formation between the aldehyde carbon of glucose (C1) and the keto carbon of fructose (C2).
Lipids comprises a diverse range of
molecules and to some extent is a catchall for relatively water-insoluble or
nonpolarcompounds of biological origin, including
waxes,
fatty acids, fatty-acid derived
phospholipids,
sphingolipids,
glycolipids, and
terpenoids (e.g.,
retinoids and
steroids). Some lipids are linear
aliphatic molecules, while others have ring structures. Some are
aromatic, while others are not. Some are flexible, while others are rigid.
[39]
Lipids are usually made from one molecule of
glycerol combined with other molecules. In
triglycerides, the main group of bulk lipids, there is one molecule of glycerol and three
fatty acids. Fatty acids are considered the monomer in that case, and may be
saturated (no
double bonds in the carbon chain) or unsaturated (one or more double bonds in the carbon chain).
[40]
Most lipids have some
polar character in addition to being largely nonpolar. In general, the bulk of their structure is nonpolar or
hydrophobic ("water-fearing"), meaning that it does not interact well with polar solvents like water. Another part of their structure is polar or
hydrophilic ("water-loving") and will tend to associate with polar solvents like water. This makes them
amphiphilic molecules (having both hydrophobic and hydrophilic portions). In the case of
cholesterol, the polar group is a mere -OH (hydroxyl or alcohol). In the case of phospholipids, the polar groups are considerably larger and more polar, as described below.
[41]
Proteins[edit]
The general structure of an α-amino acid, with the
amino group on the left and the
carboxyl group on the right.
Proteins are very large molecules – macro-biopolymers – made from monomers called
amino acids. An amino acid consists of a carbon atom attached to an
amino group, —NH
2, a
carboxylic acid group, —COOH (although these exist as —NH
3+ and —COO
− under physiologic conditions), a simple hydrogen atom, and a side chain commonly denoted as "—R". The side chain "R" is different for each amino acid of which there are 20
standard ones. It is this "R" group that made each amino acid different, and the properties of the side-chains greatly influence the overall
three-dimensional conformation of a protein. Some amino acids have functions by themselves or in a modified form; for instance,
glutamate functions as an important
neurotransmitter. Amino acids can be joined via a
peptide bond. In this
dehydration synthesis, a water molecule is removed and the peptide bond connects the nitrogen of one amino acid's amino group to the carbon of the other's carboxylic acid group. The resulting molecule is called a
dipeptide, and short stretches of amino acids (usually, fewer than thirty) are called
peptides or polypeptides. Longer stretches merit the title
proteins. As an example, the important blood
serum protein
albumin contains 585 amino acid residues.
[42]
Nucleic acids[edit]
Nucleic acids, so called because of their prevalence in cellular
nuclei, is the generic name of the family of biopolymers. They are complex, high-molecular-weight biochemical macromolecules that can convey
genetic information in all living cells and viruses.
[2] The monomers are called
nucleotides, and each consists of three components: a nitrogenous heterocyclic
base (either a
purine or a
pyrimidine), a pentose sugar, and a
phosphate group.
[47]
Structural elements of common nucleic acid constituents. Because they contain at least one phosphate group, the compounds marked
nucleoside monophosphate,
nucleoside diphosphate and
nucleoside triphosphate are all nucleotides (not simply phosphate-lacking
nucleosides).
The most common nucleic acids are
deoxyribonucleic acid (DNA) and
ribonucleic acid (RNA).
[48] The
phosphate groupand the sugar of each nucleotide bond with each other to form the backbone of the nucleic acid, while the sequence of nitrogenous bases stores the information. The most common nitrogenous bases are
adenine,
cytosine,
guanine,
thymine, and
uracil. The
nitrogenous bases of each strand of a nucleic acid will form
hydrogen bonds with certain other nitrogenous bases in a complementary strand of nucleic acid (similar to a zipper). Adenine binds with thymine and uracil; thymine binds only with adenine; and cytosine and guanine can bind only with one another.
Aside from the genetic material of the cell, nucleic acids often play a role as
second messengers, as well as forming the base molecule for
adenosine triphosphate (ATP), the primary energy-carrier molecule found in all living organisms.
[49] Also, the nitrogenous bases possible in the two nucleic acids are different: adenine, cytosine, and guanine occur in both RNA and DNA, while thymine occurs only in DNA and uracil occurs in RNA.
Metabolism[edit]
Carbohydrates as energy source[edit]
Glucose is an energy source in most life forms. For instance, polysaccharides are broken down into their monomers (
glycogen phosphorylase removes glucose residues from glycogen). Disaccharides like lactose or sucrose are cleaved into their two component monosaccharides.
Glycolysis (anaerobic)[edit]
The metabolic pathway of glycolysis converts glucose to pyruvate by via a series of intermediate metabolites. Each chemical modification (red box) is performed by a different enzyme. Steps 1 and 3 consume ATP (blue) and steps 7 and 10 produce ATP (yellow). Since steps 6-10 occur twice per glucose molecule, this leads to a net production of ATP.
|
Glucose is mainly metabolized by a very important ten-step
pathway called
glycolysis, the net result of which is to break down one molecule of glucose into two molecules of
pyruvate. This also produces a net two molecules of
ATP, the energy currency of cells, along with two reducing equivalents of converting
NAD+ (nicotinamide adenine dinucleotide: oxidised form) to NADH (nicotinamide adenine dinucleotide: reduced form). This does not require oxygen; if no oxygen is available (or the cell cannot use oxygen), the NAD is restored by converting the pyruvate to
lactate (lactic acid) (e.g., in humans) or to
ethanol plus carbon dioxide (e.g., in
yeast). Other monosaccharides like galactose and fructose can be converted into intermediates of the glycolytic pathway.
[50]
Aerobic[edit]
In
aerobic cells with sufficient oxygen, as in most human cells, the pyruvate is further metabolized. It is irreversibly converted to
acetyl-CoA, giving off one carbon atom as the waste product
carbon dioxide, generating another reducing equivalent as
NADH. The two molecules acetyl-CoA (from one molecule of glucose) then enter the
citric acid cycle, producing two more molecules of ATP, six more NADH molecules and two reduced (ubi)quinones (via
FADH2 as enzyme-bound cofactor), and releasing the remaining carbon atoms as carbon dioxide. The produced NADH and quinol molecules then feed into the enzyme complexes of the respiratory chain, an
electron transport system transferring the electrons ultimately to oxygen and conserving the released energy in the form of a proton gradient over a membrane (
inner mitochondrial membrane in eukaryotes). Thus, oxygen is reduced to water and the original electron acceptors NAD
+ and
quinone are regenerated. This is why humans breathe in oxygen and breathe out carbon dioxide. The energy released from transferring the electrons from high-energy states in NADH and quinol is conserved first as proton gradient and converted to ATP via ATP synthase. This generates an additional
28 molecules of ATP (24 from the 8 NADH + 4 from the 2 quinols), totaling to 32 molecules of ATP conserved per degraded glucose (two from glycolysis + two from the citrate cycle).
[51] It is clear that using oxygen to completely oxidize glucose provides an organism with far more energy than any oxygen-independent metabolic feature, and this is thought to be the reason why complex life appeared only after Earth's atmosphere accumulated large amounts of oxygen.
Gluconeogenesis[edit]
In
vertebrates, vigorously contracting
skeletal muscles (during weightlifting or sprinting, for example) do not receive enough oxygen to meet the energy demand, and so they shift to
anaerobic metabolism, converting glucose to lactate. The
liver regenerates the glucose, using a process called
gluconeogenesis. This process is not quite the opposite of glycolysis, and actually requires three times the amount of energy gained from glycolysis (six molecules of ATP are used, compared to the two gained in glycolysis). Analogous to the above reactions, the glucose produced can then undergo glycolysis in tissues that need energy, be stored as glycogen (or
starch in plants), or be converted to other monosaccharides or joined into di- or oligosaccharides. The combined pathways of glycolysis during exercise, lactate's crossing via the bloodstream to the liver, subsequent gluconeogenesis and release of glucose into the bloodstream is called the
Cori cycle.
[52]
Relationship to other "molecular-scale" biological sciences[edit]
Researchers in biochemistry use specific techniques native to biochemistry, but increasingly combine these with techniques and ideas developed in the fields of
genetics,
molecular biology and
biophysics. There has never been a hard-line among these disciplines in terms of content and technique. Today, the terms
molecular biology and
biochemistry are nearly interchangeable. The following figure is a schematic that depicts one possible view of the relationship between the fields:
- Biochemistry is the study of the chemical substances and vital processes occurring in living organisms. Biochemists focus heavily on the role, function, and structure of biomolecules. The study of the chemistry behind biological processes and the synthesis of biologically active molecules are examples of biochemistry.
- Genetics is the study of the effect of genetic differences on organisms. Often this can be inferred by the absence of a normal component (e.g., one gene), in the study of "mutants" – organisms with a changed gene that leads to the organism being different with respect to the so-called "wild type" or normal phenotype. Genetic interactions (epistasis) can often confound simple interpretations of such "knock-out" or "knock-in" studies.
- Molecular biology is the study of molecular underpinnings of the process of replication, transcription and translation of the genetic material. The central dogma of molecular biology where genetic material is transcribed into RNA and then translated into protein, despite being an oversimplified picture of molecular biology, still provides a good starting point for understanding the field. This picture, however, is undergoing revision in light of emerging novel roles for RNA.[53]
- Chemical biology seeks to develop new tools based on small molecules that allow minimal perturbation of biological systems while providing detailed information about their function. Further, chemical biology employs biological systems to create non-natural hybrids between biomolecules and synthetic devices (for example emptied viral capsids that can deliver gene therapy or drug molecules).[54]